Engineered endosymbiosis: what would be some amazing collaborations?
Image credit: National Institutes of Health (NIH)
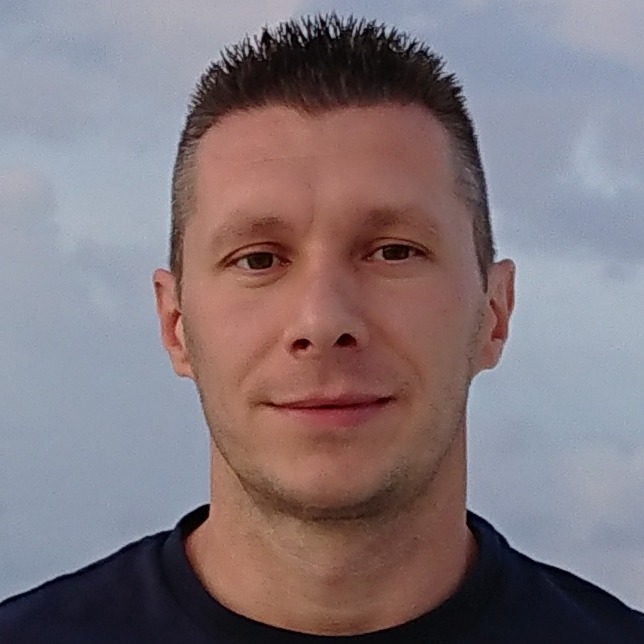
Please leave the feedback on this challenge
Necessity
Is the problem still unsolved?
Conciseness
Is it concisely described?
Bounty for the best solution
Provide a bounty for the best solution
Bounties attract serious brainpower to the challenge.
[1]https://en.wikipedia.org/wiki/Symbiogenesis
Creative contributions
Mimic endosymbiosis: models and example
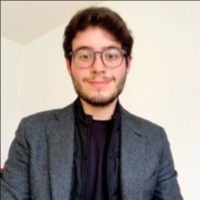
[1]Engineering yeast endosymbionts as a step toward the evolution of mitochondria Angad P. Mehta, Lubica Supekova, Jian-Hua Chen, Kersi Pestonjamasp, PaulWebster, Yeonjin Ko, Scott C. Henderson, Gerry McDermott, FrantisekSupek, Peter G. Schultz
[2]Ludington WB, Ja WW (2020) Drosophila as a model for the gut microbiome. PLoS Pathog 16(4): e1008398. https://doi.org/10.1371/journal.ppat.1008398
[3]Nigro G, Hanson M, Fevre C, Lecuit M, Sansonetti PJ. Intestinal Organoids as a Novel Tool to Study Microbes-Epithelium Interactions. Methods Mol Biol. 2019;1576:183-194. doi: 10.1007/7651_2016_12. PMID: 27628134.
[4]Chowdhury, S., Castro, S., Coker, C. et al. Programmable bacteria induce durable tumor regression and systemic antitumor immunity. Nat Med 25, 1057–1063 (2019). https://doi.org/10.1038/s41591-019-0498-z
Please leave the feedback on this idea
Nitrogen fixing bacteria within our skin cells
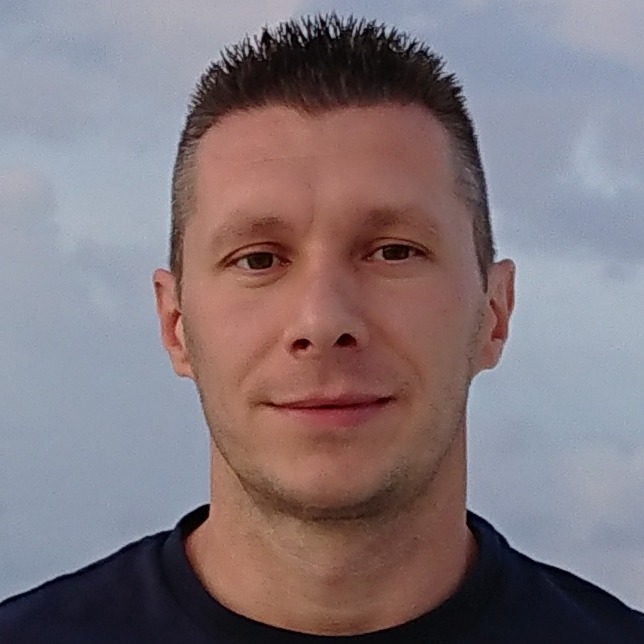
Please leave the feedback on this idea
Endosymbiosis engineering for containing anti-oxidant producing bacterial species
[1] Schieber M., Chandel N.S. ROS function in redox signaling and oxidative stress. Curr. Biol. 2014;24:R453–R462. doi: 10.1016/j.cub.2014.03.034
[2]Luo D., Fang B. Structural identification of ginseng polysaccharides and testing of their antioxidant activities. Carbohydr. Polym. 2008;72:376–381. doi: 10.1016/j.carbpol.2007.09.006.
[3]Lin, X., Xia, Y., Wang, G., Yang, Y., Xiong, Z., Lv, F., Zhou, W., & Ai, L. (2018). Lactic Acid Bacteria With Antioxidant Activities Alleviating Oxidized Oil Induced Hepatic Injury in Mice. Frontiers in Microbiology, 9, 1–17. https://doi.org/10.3389/fmicb.2018.02684
Please leave the feedback on this idea
Make the symbiont's DNA incomplete without the host cell's DNA
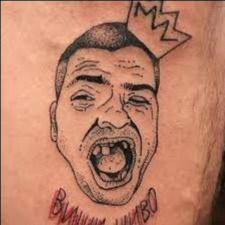
Please leave the feedback on this idea
What causes parts of symbiont's DNA to move to the host cell's nucleus?
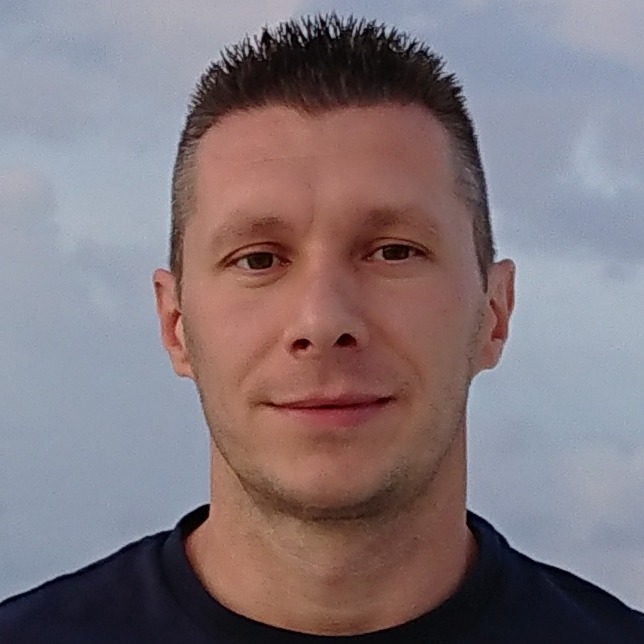
[1]https://microbewiki.kenyon.edu/index.php/Aphids_and_Buchnera
[2]https://en.wikipedia.org/wiki/Blue-ringed_octopus
Please leave the feedback on this idea
Cyanobacteria + nanoparticles + near-infrared light = human photosynthesis
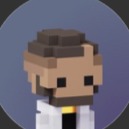
[1]https://pubs.acs.org/doi/10.1021/acs.nanolett.1c00719
Please leave the feedback on this idea
Microalgae with antibiotic-loaded polymeric nanoparticles coated with a neutrophil membrane? Done!
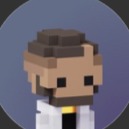
[1]https://www.nature.com/articles/s41563-022-01360-9
Please leave the feedback on this idea
Magnetosomes from Magnetotactic bacteria
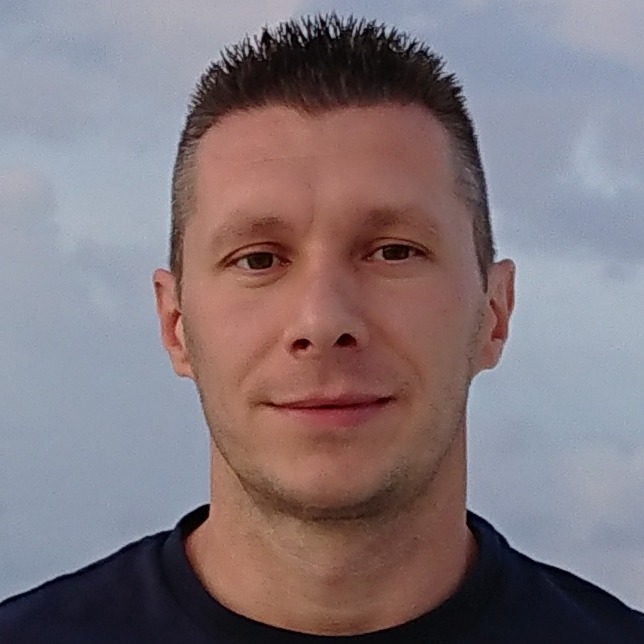
Please leave the feedback on this idea
Deinococcus radiodurans as safe-keeper of the host cell's DNA
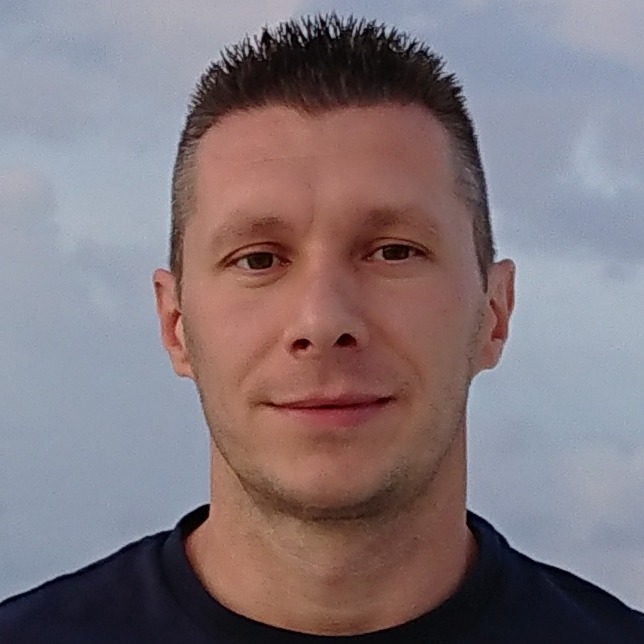
[1]Minton KW. DNA repair in the extremely radioresistant bacterium Deinococcus radiodurans. Mol Microbiol. 1994 Jul;13(1):9-15. doi: 10.1111/j.1365-2958.1994.tb00397.x. PMID: 7984097.
Please leave the feedback on this idea
Add your creative contribution
General comments
Please leave the feedback on this idea
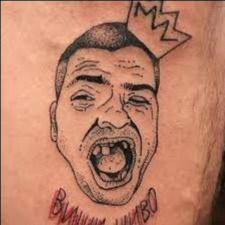
Please leave the feedback on this idea
Please leave the feedback on this idea
Please leave the feedback on this idea